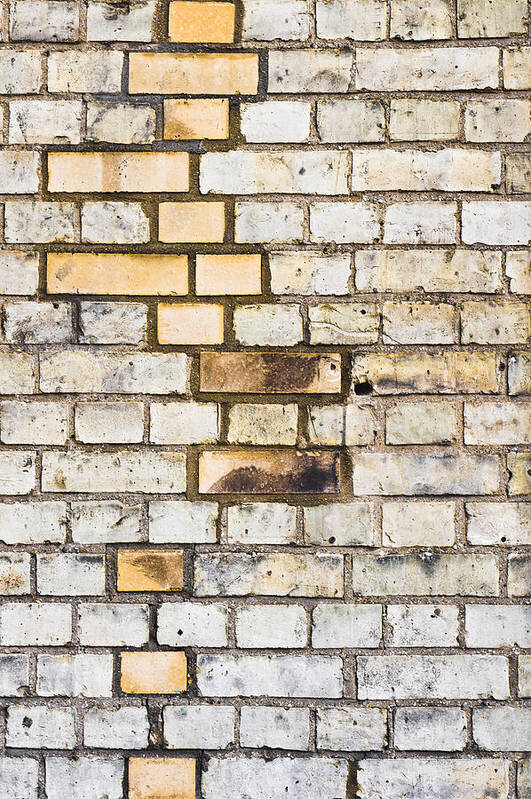
Bricks
Two of the most important challenges facing the renewable energy transition are urban growth and peak demand. Urban growth here refers to the physical growth of urban environments - specifically, the need for constructing more and more buildings, either to house more people or provide workspaces for business growth. Peak demand refers to the unequal distribution of energy use throughout the day. Energy demand spikes in the mornings and evenings, while it dips a bit during work hours and drops significantly overnight. This presents a huge problem for renewable energy systems, as their energy production is limited by the availability of the resource, whereas fossil fuels can be loaded as needed (Hydro and biofuel can provide peak demand, but hydroelectric potential is limited around the world and biofuels are resource-intensive). The obvious solution is to store excess energy in batteries, but large enough systems are extremely expensive. While these two challenges are pressing, they have traditionally been decidedly unrelated issues. If anything, they are almost at odds with each other. However, recent research has reshaped this perspective: buildings may be the answer to energy storage.
Researchers at Washington University of St. Louis have recently developed a way to turn bricks into the energy storage devices we need to solve the peak demand issue [1]. Yes, the plain ol’ 65 cent red bricks from the local hardware store. See, bricks contain a compound called iron oxide - this is what gives them their red color. It is also reactive to a number of different compounds, and the research team at WUSTL reacted the iron oxide with HCl and EDOT (ethylenedioxythiophene) to produce PEDOT (polyethylenedioxythiophene), an electrically conductive polymer [1]. Putting two of these bricks together using an electrolytic gel creates a supercapacitor. The researchers find that a brick wall constructed using this technique would have a capacitance density of 11.5 kF/m^2 and a power density of 1.61 W/m^2 [1]. In practical terms, they say that 50 bricks could power emergency lighting for a house for five hours [2].
While supercapacitors can not supply power as long as batteries can (hours vs. days), this issue is not particularly important when considering peak demand, as peak demand only lasts for a few hours each day. Both should be equally effective at lasting as long as it needs to. The advantage of supercapacitors, though, is that they can be (dis)charged much faster, allowing them, for example, to respond much quicker to sudden demand spikes on the grid. Additionally, they can be cycled millions of times with almost no degradation in quality. Thus, since the production process does not affect the structural integrity or weather-resistiveness of the brick [1], maintenance costs for a larger-scale device should be extraordinarily low. Furthermore, the production process only requires one human step - the rest is “a self-assembly process” [3]. This enables production to become very cheap and easily scalable for large quantities of bricks. While this technology, like all in this series, requires significant further investment, so too did ‘conventional’ renewable energies. And almost none of them showed as much promise in cost and scalability at their outset as this one does.
An interesting implication of these `brick supercapacitors` is that they reduce our need for conventional Li-ion or Pb-acid batteries. These batteries have a much shorter lifespan than supercapacitors, so expanding the use of the bricks as a co-storage device should see an increase in lifespan for the Li-ion batteries. Moreover, lithium is only produced in a handful of sites around the world [4]. This gives these few countries huge global power in the same way fossil fuels have shaped geopolitics for the past half-century (most notably putting huge importance on relationships with the Middle East). Whereas, the manufacturing process of the ‘energy bricks’ only uses widely available chemicals and processes. It promotes energy democratization, both in the sense that it brings energy infrastructure to the people, and that it brings energy competitiveness to resource-poor and developing nations.
Think about how many bricks we use. Alongside concrete, they compose a large majority of the urban environment. In fact, there’s a good chance your house or the building you are in is made of brick. Estimates put the global production of bricks at over 1.5 billion per year [5], and output is expected to remain this level for a while as urbanization continues and people need places to live. So, while brick production does produce some black carbon emissions, fully harnessing their energy storage capacity could dramatically increase the ability of energy grids to fully switch to renewables. The conventional wisdom says that energy drain is an inherent fact of cities, but `energy bricks` demonstrate that energy storage also might be, quite literally, woven into the fabric of cities themselves.
Citations [Bricks]
[1] Wang, H., Diao, Y., Lu, Y., Yang, H., Zhou, Q., Chrulski, K., and D’Arcy, J. “Energy storing bricks for stationary PEDOT supercapacitors,” Nature Communications, 11(3882), 2020. doi:10.1038/s41467-020-17708-1
[2] Ogliore, T. “Storing energy in red bricks,” the Source, Washington University in St Louis, 2020. https://source.wustl.edu/2020/08/storing-energy-in-red-bricks. Accessed 29 Nov, 2020.
[3] Ballard, S. “Turning rust into supercapacitors,” Department of Chemistry, Washington University in St. Louis, 2020. https://chemistry.wustl.edu/news/turning-rust-into-supercapacitors. Accessed 29 Nov, 2020.
[4] Jaskula, B. “Mineral Commodity Summaries 2020,” U.S. Geological Survey, 2020.
[5] “The Brick Market,” Habla Kilns, 2019. https://www.hablakilns.com/the-brick-industry/the-brick-market/. Accessed 29 Nov, 2020.